Solar Atmosphere
While our understanding of the structure and dynamics of the solar atmosphere has increased significantly in recent years, a clear view of the mechanisms that heat the atmosphere and transport energy and momentum through it still eludes us. To address this issue we study the morphology and dynamics of the solar atmosphere using the magneto-acoustic-gravity waves that are prevalent throughout the atmosphere. Our current research focuses on studying the Sun’s (atmospheric) internal gravity waves. These waves can transport energy and momentum over large distances and are likely to play a significant role in the dynamics of the atmosphere. Their oblique propagation and small vertical wavelengths have made them challenging to observe and, consequently, little is known about them. Our group has succeeded in measuring these waves (Calchetti et al. 2020) and now we are taking steps toward capitalizing on their potential as a diagnostic tool for probing the solar atmosphere (the root of space weather).
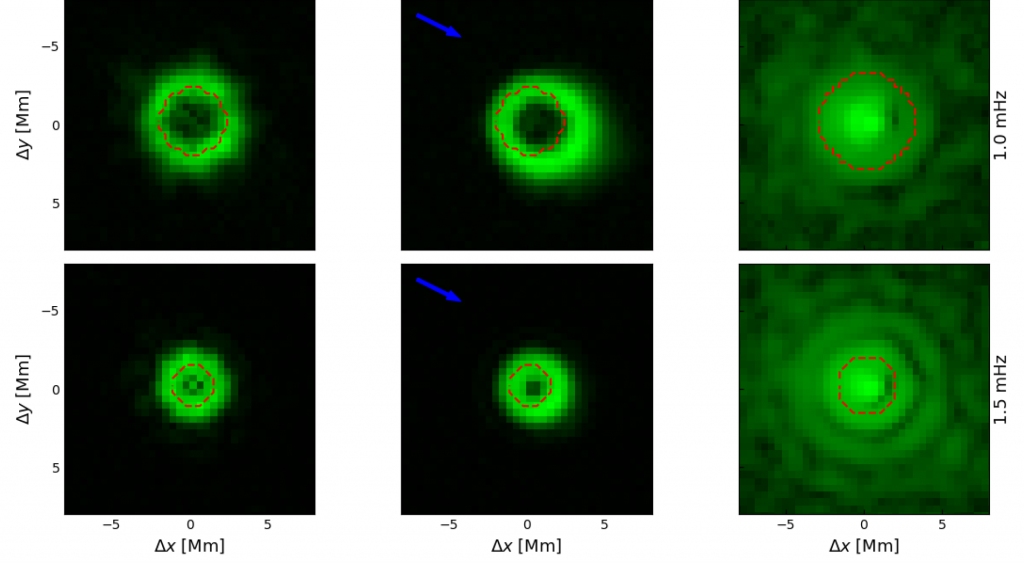
Our group has also recently used acoustic waves to make the first spatial maps of the acoustic cut-off frequency, sound speed, and radiative damping in the solar atmosphere (Jefferies et al. 2019, Fleck et al. 2020). These fundamental quantities are important for understanding the mechanical heating and wave behavior in the solar atmosphere.
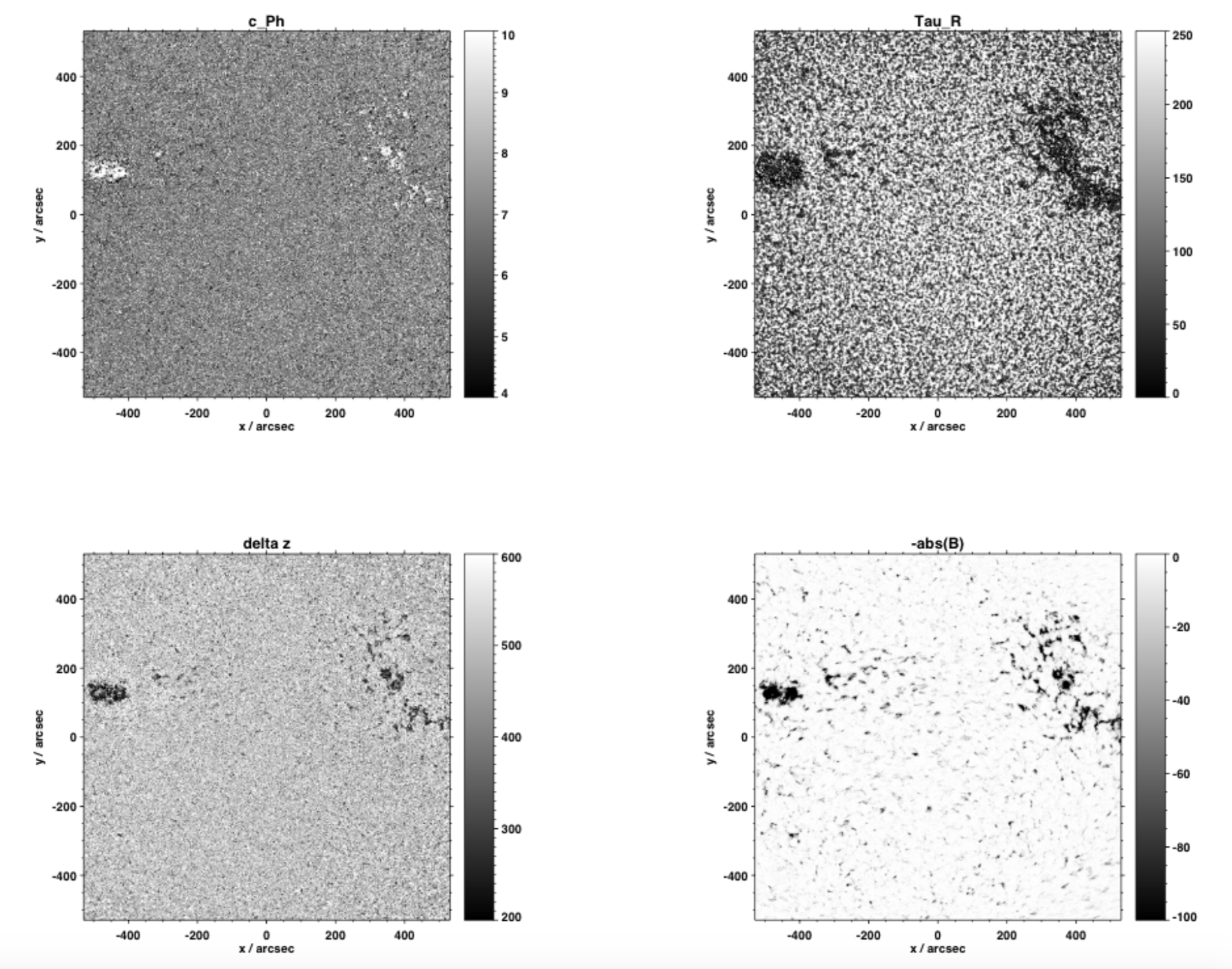
Solar Interior: Near Surface Layers
Local helioseismology uses the acoustic wave field observed at the solar surface to infer sub-surface structure and flows. It is also used to detect the presence of magnetic regions on the far side of the Sun. Recent research has shown that many of the inferences we have made using this technique are affected by systematic effects related to the height in the solar atmosphere at which the observations were made, significantly undermining the value of local helioseismology. We are currently looking at the use of the multi-height velocity and magnetic data from our MOTH II Doppler-magnetographs to separate the internal and atmospheric contributions to the measured acoustic wave travel times, so that we can determine reliable information on the Sun’s sub-surface structure and dynamics (flows, etc.).
Solar Interior: Tachocline and solar core
We are engaged in research to accurately characterize the nature and temporal variation of the tachocline region in the solar interior (believed to be the location of the solar dynamo). Detailed determinations of the rotation and asphericity profiles in the vicinity of the solar tachocline, and their variation in time, will provide important constraints for solar dynamo models. This, in turn, will contribute to a better understanding of heliospheric dynamics.
Currently, reliable assessment of the flows and properties of the solar tachocline (i.e. the existence of jets or tachocline oscillations) is impeded by large systematic errors that presently exist in the fitting of the spectral peaks in the power spectrum of the Sun’s low- and intermediate-degree p modes. Along with Sergei Vorontsov (Queen Mary College, University of London) we have developed procedures to make the first steps in replacing this process, and the subsequent inversion of the fitted p-mode parameters, with a single procedure that will directly fit the observed oscillation spectra with model spectra determined through a parameterization of a rotating aspherical seismic solar model.
We are also involved in the development of two space missions to directly measure small-scale flows on and below the tachocline: Solar Activity FAR side Investigation (SAFARI) and Solar Activity Investigation (SAI). Both projects are spear headed by the Jet Propulsion Laboratory. SAFARI will explore the solar interior and understand how magnetic fields develop there and emerge to the solar surface. In particular, SAFARI will measure the morphology and dynamics of the deep solar interior (the core). This will be achieved by viewing the Sun from two widely separated viewing points – a satellite in an Earth-trailing orbit and a global network of ground-based instruments – and using low-degree solar seismic waves to probe the deep solar interior.
The SAI mission is designed to act as a precursor for the SAFARI mission and uses a 6U cubesat version of the SAFARI instrument. SAI will be launched in an Earth-leading orbit and will use the HMI instrument in Earth orbit for the second viewing point. The science target for this mission is to explore the origins of solar and heliospheric variability by probing the deep interior of the sun and tracking the evolution of magnetic activity.
Doppler and magnetic observations from widely separated viewing points are expected to be a key component of a future space weather-monitoring constellation. Our work on cubesat scale instrumentation, and the necessary cubesat capabilities (communications and accurate pointing, for example) will pave the way for the deployment of such a constellation.